Superconductivity: Boiling temperature effects 24
Superconductivity
For more than a century, scientists and engineers have been fascinated by the extraordinary quantum phenomenon known as superconductivity. Its special qualities—like its zero electrical resistance and ability to expel magnetic fields—have opened the door for ground-breaking uses in a variety of industries, including transportation, quantum computing, and medical imaging.
The foundations of superconductivity, Cooper pairs, the Meissner effect, the BCS theory, and the critical temperature (Tc) will all be covered in this article.
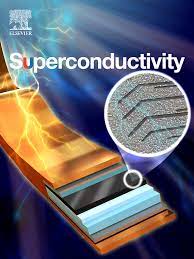
What is superconductivity?
A state of matter known as superconductivity allows a substance to carry electricity with no electrical resistance. This implies that there is no energy loss during the infinite flow of an electrical current through a superconductor. Ordinary electrical conductivity causes materials to exhibit some resistance, which results in the release of energy as heat. This feature stands in striking contrast to that.
Differences from Ordinary Electrical Conductivity
Because of collisions between the electrons and the atomic lattice of ordinary conductors like copper or aluminum, electric current cannot pass through them. Heat is produced and energy is lost in these crashes. However, in superconductors, electrons form Cooper pairs, which flow through the lattice without scattering and provide zero resistance.
What is the Meissner Effect?
The phenomenon known as the Meissner effect occurs when a superconductor below its critical temperature (Tc) enters the superconducting state and expels all magnetic fields from its core. A distinguishing feature of superconductivity, this effect sets it apart from perfect conductivity, which does not always result in the removal of magnetic fields.
What are Cooper pairs?
Electron couples known as cooper pairs flow through superconducting materials without scattering off the atomic lattice. These pairs arise as a result of an attractive relationship mediated by phonons, which are vibrations inside the lattice. Because they have opposing spins and momenta, the electrons in a Cooper pair can join in a fashion that saves energy and avoids scattering.
Please follow Renewable Energy for allied topics.
Contribution of Cooper Pairs to Superconductivity.
Since they allow electrons to flow through the lattice coherently and without resistance, cooper pairs are crucial to superconductivity. The electronic density of states at the Fermi level experiences an energy gap due to Cooper pair production, which inhibits scattering and hence removes electrical resistance. Superconductors have a special zero-resistance feature because of this synchronized movement of electron pairs.
How Does the BCS Theory Explain Superconductivity?
Superconductivity in typical superconductors is explained in detail by the BCS theory, which bears the names of its creators, Bardeen, Cooper, and Schrieffer. As per the BCS hypothesis, the emergence of superconductivity is attributed to the condensation of Cooper pairs into a collective quantum ground state.
There is no electrical resistance in this condition because the electrons flow through the material coherently and without scattering. A critical component of preserving the superconducting state is the energy gap that develops at the Fermi level, which is also explained by the BCS theory. New superconducting materials have been found thanks to this hypothesis, which has also been crucial in understanding the microscopic principles behind superconductivity.
What is the Critical Temperature (Tc)?
The temperature below which a material enters the superconducting state is known as the critical temperature (Tc). The material returns to its usual conductive condition with resistance when the temperature rises above because there is enough thermal energy to break the Cooper pairs.
What is the importance of the critical temperature?
The critical temperature is an important parameter in superconductivity because it establishes boundaries with superconducting materials. Due to their higher Tc values, high-temperature superconductors (HTS) are more suitable for applications requiring less intense cooling. Conversely, low-temperature superconductors (LTS) have lower Tc values and necessitate more advanced cooling techniques.
Superconductivity research is primarily focused on understanding and finding materials with higher Tc values because doing so could result in more widely used and economically viable uses of superconducting technology.
What happens when a superconductor heated to boiling temperatures?
Superconductors go through a phase transition and lose their superconducting characteristics when they are heated above their critical temperature (Tc).
In specifics, this is what occurs:
Decrease in Electrical Resistance to Zero
Ability to carry electricity without resistance is one of the features that makes a material a superconductor. Above Tc, the material returns to a normal conductive state and displays electrical resistance similar to that of any other regular conductor. When electron pairs that go through the lattice without scattering, known as Cooper pairs, break apart, the resistance resurfaces.
The Meissner Effect vanishes
Magnetic fields are ejected from the superconductor’s interior, a phenomenon known as the Meissner effect. Beyond Tc, the material becomes unable to block magnetic fields and takes on the characteristics of a typical conductor, allowing magnetic fields to pass through.
Phase Changeover
The electronic structure of the material changes during the phase transition from a superconducting to a normal state. Electrons form Cooper pairs in the superconducting state and condense into a collective ground state. These Cooper pairs are broken by thermal energy as the temperature rises over Tc, returning the electrons to an unpaired, typical metallic state.
Effects on Applications
The gadget would fail if the critical temperature were exceeded in practical applications such MRI machines, maglev trains, or superconducting magnets. Maintaining superconducting characteristics and ensuring the effective functioning of such devices requires keeping superconductors below Tc.
Energy Gaps exist
An energy gap exists in the electronic density of states at the Fermi level in the superconducting state. As temperature increases above Tc, the gap closes and electrons move into higher energy states due to the breaking of Cooper pairs.
So, we see that heating a superconductor above its critical temperature eliminates the material’s special qualities, such as zero electrical resistance and the Meissner effect, and returns it to its regular, non-superconducting state.
What are the uses of superconductivity?
Some of the most common uses of Superconductivity are as follows:
Imaging and Diagnostics in Medicine
Magnetic Resonance Imaging, or MRI
Magnetic Resonance imaging (MRI) is one of the most well-known uses of superconducting. Superconducting magnets are used in MRI scanners to create the powerful, stable magnetic fields required to produce finely detailed images of the interior components of the human body. High picture resolution and quick imaging times are guaranteed by the employment of superconducting magnets.
Benefits
High-quality pictures, reduced scan times, and lower energy usage.
Transmission of Power
Power networks can benefit greatly from superconducting wires since they can carry electricity with almost no energy loss. Higher current densities may be carried by these cables than by traditional copper or aluminum wires.
Advantages
Less energy loss , More capability for existing Lighter and smaller cables are required. Decreased energy usage
Magnets with superconductivity
Superconducting magnets find application in fusion reactors and particle accelerators, among other things. These magnets have the capacity to provide the incredibly powerful magnetic fields required for fusion reactor plasma confinement or accelerator particle acceleration to high energies.
Elevated strength of the magnetic field; enhanced productivity
What is the main cause of superconductivity?
Superconductivity above zero temperature is mostly caused by quantum fluctuation, which is explained by the short-range super force field, which also explains the quantum phase shift at zero temperature.
What destroys superconductivity?
By applying an increased magnetic field, one can disrupt superconductivity. This causes the material to be penetrated and suppresses the Meissner effect, which is a phenomenon of field expulsion.
Who is the father of superconductivity?
Heike Kamerlingh Onnes was the first to produce liquid helium in 1908, which directly contributed to his superconductivity discovery in 1911. The person who discovered superconductivity is Heike Kamerlingh Onnes (on the right).